Sign up for daily news updates from CleanTechnica on email. Or follow us on Google News!
Supercritical CO2 is having a moment in the sun again, at least from my admittedly odd point of view. The US DOE is once again touting the use of the substance in thermal electrical generation. The carbon capture and sequestration crowd are touting supercritical CO2 pipelines as the answer to the reality that burning fossil fuels makes CO2 that’s 2-3 times the mass and 450 times or more the volume of the fuels. And it’s being used in textiles and to extract interesting herbal elements from high-value plants that are legal in many jurisdictions. Heck, it’s even in heat pumps.
Phases Of Matter
There’s a lot to unpack here, so lets head back to the 19th century to figure out what the heck supercritical CO2 is. No, lets head back further to the ancient Greeks. And to be clear, it’s entirely probable that the ancient Chinese and ancient Arabians figured this out independently and possibly before Europeans did, but English-language histories were written by Europeans, so we’ll live with this version of the story. (If anyone has references to non-European scientists and phases of matter, please let me know. I love good parallel invention stories.)
Humans have known pretty much forever that water and ice are the same thing, with the difference between them being heat. Summer and winter with icing and melting are pretty obvious phenomena, and humans have been smart enough to remember and figure things out for probably 450,000 years. Gases took a lot longer to understand as another state of matter. The Greeks knew this and mistakenly thought that with water being able to exist in three states, everything in the universe was probably made of it.
It takes a lot of heat to change the phase of ice to water. The temperature of ice or any solid rises until it gets to the point where it’s going to turn to a liquid, and then it just sits there at that temperature as more and more heat is added until finally it becomes a liquid. Ditto for water turning to steam. Water gets to the boiling point and doesn’t get hotter. It sits there at that temperature for a long time, soaking up more heat, until the phase change starts happening and steam starts bubbling off.
A lot of early physics assumed that the increase in temperature was linear between ice, water, and steam and a lot of physics was rewritten when sensible people started actually measuring it. So we have three states of matter, and the energy is an indicator that something unusual is going on. Scientists love unusual things, engineers not so much.
And scientists also figured out that pressure had a lot to do with it. Higher pressures meant that water turned into steam only at much higher temperatures. Ditto the reverse, so anyone trying to make a hot cup of tea at the top of Mt. Everest gets a lukewarm cup instead. And there was a critical temperature above which no amount of pressure will force a gas to turn into a liquid and that was discovered as well.
A bunch of work had to do with ideal gases vs real ones, which is exactly what it sounds like. An ideal gas is a hypothetical mathematical construct plugged into equations for various purposes. A real gas is one that actually exists and has particles and forces within and between the particles. Messy reality again.
Supercritical Matter
That something weird was going on was observed in the early 19th century when a Baron was doing experiments and found the oddity, but it wasn’t explained or explicable until later. Similarly, chemist and physicist Thomas Andrews did a bunch of experiments that resulted in supercritical fluids in the late 19th century.
In the same period, from about 1820 to 1873, a bunch of very interesting experiments, theory, and math was done. Gibbs, Maxwell, and van der Waals, giants of physics, were all poking at this, doing experiments, creating theories and testing equations. Van der Waals ended up with an equation in 1873 that suggested that there was a fourth state of matter that was both a gas and a liquid below the critical temperature. True for every form of matter, by the way. That won van der Waals the Nobel Prize in 1910.
That fourth state of matter that’s both liquid and gas is the supercritical state. When we talk about supercritical CO2, we’re talking about CO2 that’s been brought carefully to the right temperature and pressure combination to shift into this weird state of matter.
Phase Diagrams
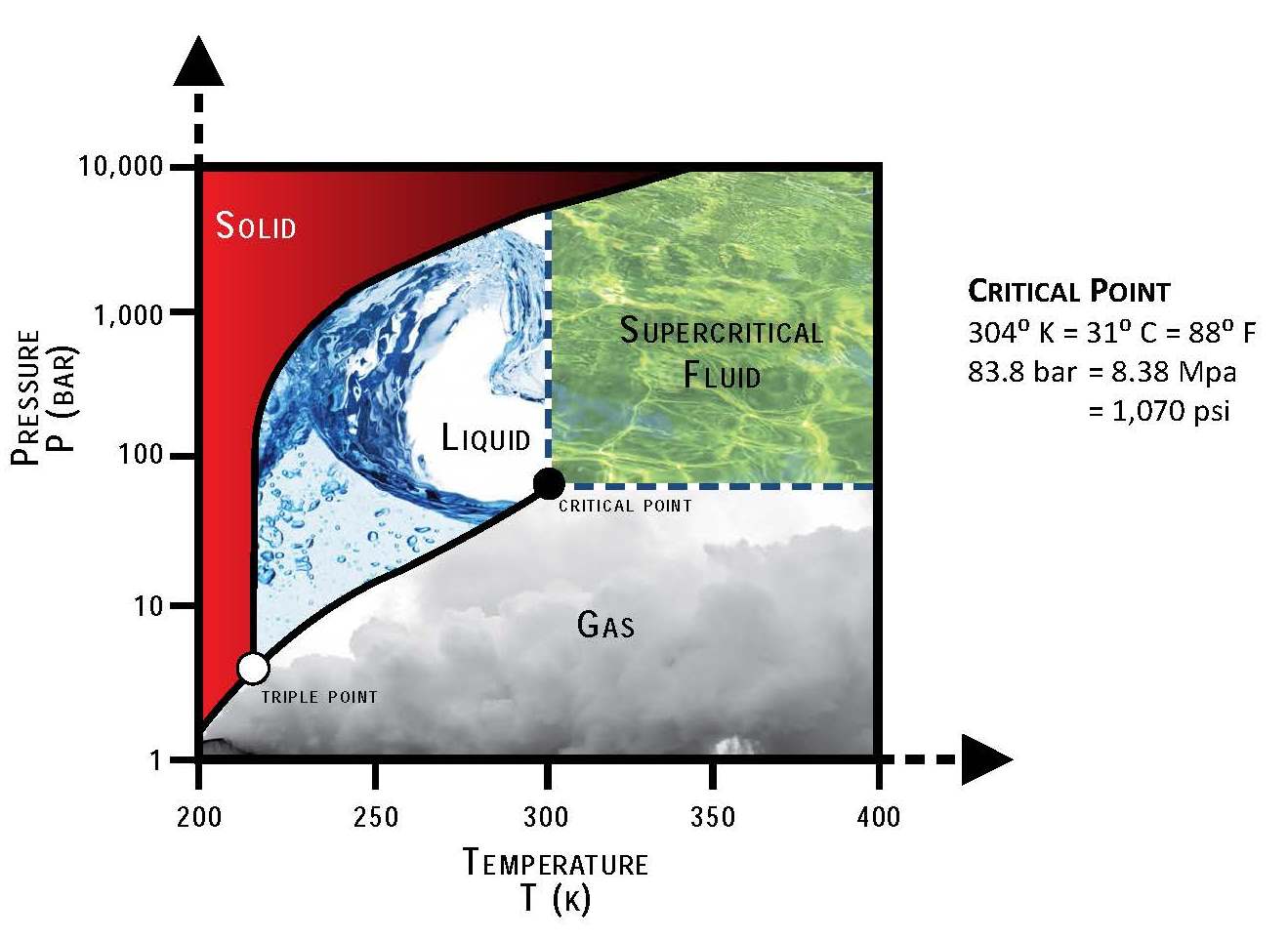
Phase diagram for CO2 from US Department of Energy
Lots of experimentation and quantification ensued. Lots of phase diagrams, like the one for CO2 above, were created. A phase diagram just says that as temperature and pressure slowly change, a specific form of matter will move into a new state, and what that state is. If they are solutions or alloys, the phase diagrams are different than for the matter that makes them up, often profoundly different in very weird and useful ways. Rare earths make alloys with other metals that enable rather absurd percentages of our modern electronic, electrical, and digital technologies, for example.
There’s actually a third dimension to this chart which exists in tables. It’s the dimension of density of the supercritical CO2. As you move upward to the right, supercritical CO2 actually gets denser. That’s why the diagram has 83.8 bar (almost exactly one atmosphere at sea level, but in metric), because it’s a bit higher pressure. The density can change quite radically with increases, so that at about three times the pressure and the same temperature, it can go from 161 kg per cubic meter to 941 kg per cubic meter. It behaves like a gas in that regard, but much denser.
Engineers were suddenly happy because the weird stuff had explanations, and when asked they could design kit to shift matter into this state and maintain it in that state. Far from trivial work, by the way, but engineering, not figuring out what the heck was going on, establishing a theory and math for it, finding out that the theory and math were wrong a thousand times and finally getting theory, math and experiments all agreeing without any fudging. Or at least not much, because there’s always quantum physics and the special theory of relativity to mess things up.
The question became, what was this stuff good for? There are places where it exists naturally, like around thermal vents at the bottom of the ocean, but in general it seemed more like an oddity than something exploitable. But like 3M’s famous weak glue that spawned billions of Post-It Notes, around 1970 people started figuring it out.
How Do We Make Supercritical CO2?
But before we get to what it’s used for, let’s talk about how it’s made and maintained. That’s important, as you’ll see.
To get supercritical CO2, you have to slowly raise both the pressure and temperature until it gets to that supercritical point. That’s done by slowly increasing the pressure with energy. As the pressure of most gases increases, they get hotter. Similarly, as the pressure decreases they get colder. That’s true for CO2. It’s not true for hydrogen, which gets hotter as it expands, one of the many, many thermal challenges with managing hydrogen that makes grown engineers gnash their teeth and pull out their hair.
That increase in heat means you have to remove some of the heat. Then you have to repeat the cycle. You have to get CO2 up to 74 atmospheres and 31° Celsius. The pressure is the equivalent of being about 6.4 meters or about 21 feet under water. It’s not extreme, but it’s not trivial. The optimal number of compression cycles from an energy efficiency perspective turns out to be six to seven. That gets the initial creation of supercritical CO2 to 90 kWh per ton of the substance, assuming room temperature CO2 at normal air pressure.
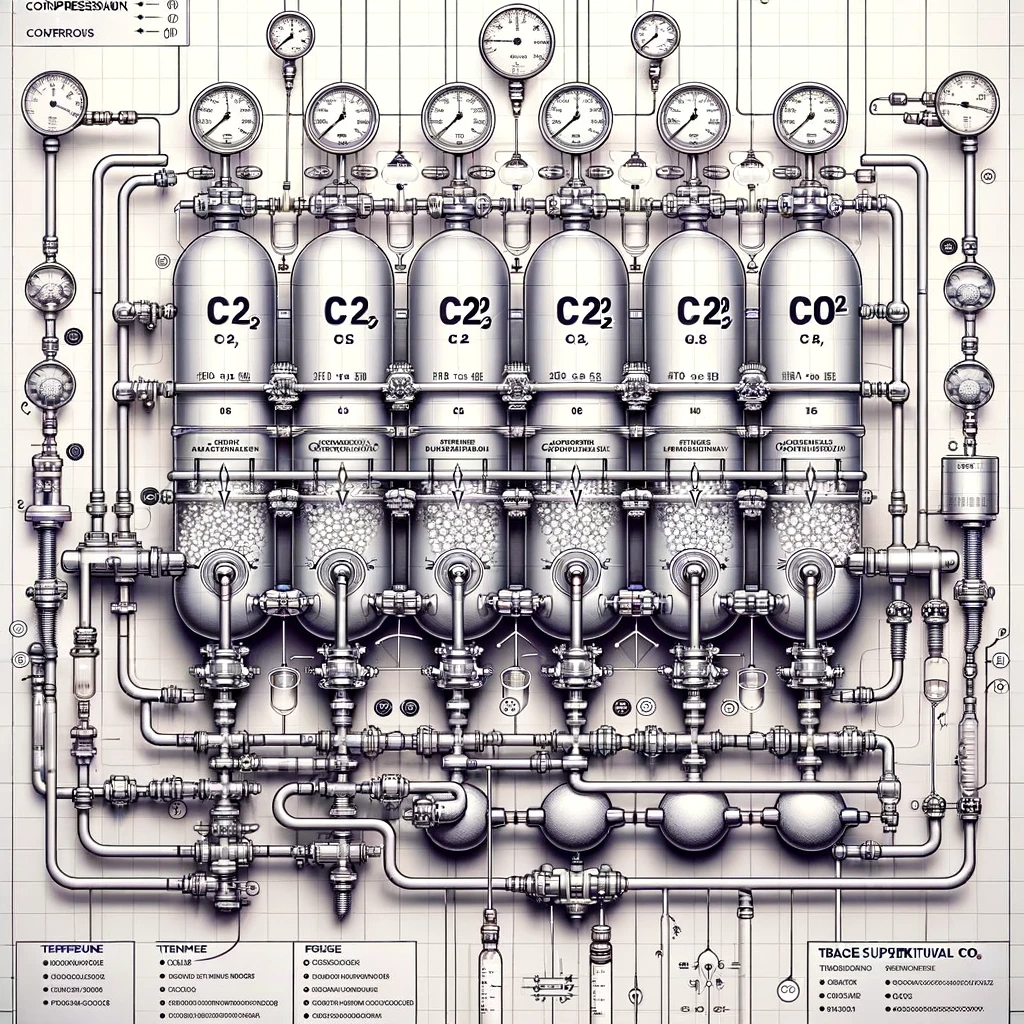
ChatGPT and DALL-E generated image of a schematic of a six-stage CO2 compression device designed to produce supercritical CO2.
If you don’t care as much about energy efficiency, you can use fewer cycles and more refrigeration, and there are alternative technologies that can be used for compression, of course. The key takeaway is 90 kWh per ton of supercritical CO2.
And remember that bit about making it denser. Well, three times the pressure is three times the energy for compression and three times the energy for removing the heat that is being created. No free lunch with this.
What’s Supercritical CO2 Good For?
Now we get to the 1970s. Not just disco, shag carpet, and embarrassing photos of your older relatives, but actual uses for supercritical CO2, specifically coffee.
For no reason that has ever been clear to me, German scientist Kurt Zosel invented decaffeinated coffee. The process uses supercritical CO2. This is one of the cases where the gas and liquid duality of supercritical fluids becomes useful. Because it behaves like a gas, it can flow through the coffee beans. Because it behaves like a liquid, it can make a solution with the caffeine and then continue to flow out of the beans again, taking the caffeine with it. And it doesn’t take any of the aromatics that give coffee its odor and taste with it, just the caffeine. Then the CO2 turns into a gas and disappears, leaving the caffeine behind. Then, of course, people sell coffee with no caffeine to one market and put the caffeine into energy drinks for another market. Neat hack.
That odd hack is very widely used to extract various substances from various plants for repackaging. If you are thinking cannabis and herbal supplements, you are exactly correct. It’s also used widely in dry cleaning, where it’s vastly less problematic than legacy chemicals. In both of those cases, the systems tend to be closed, where the CO2 doesn’t go anywhere. It gets turned into supercritical CO2, used to extract the necessary chemicals, turned into a gas to leave the chemicals behind, then filtered to get rid of any residue, turned back into CO2, and reused.
Increasingly, supercritical CO2 is turning up in heat pumps and refrigeration units. Sanden’s CO2 hot water heat pump is actually a supercritical CO2 heat pump, leveraging the physics for very efficient heat transfer.
The volumes aren’t enormous. A big cannabinoid extraction unit might process a 1,000 kg of plant material in 24 hours, and push 22 kg of supercritical CO2 through the system every minute. A heat pump might have a kilogram of CO2.
Enhanced Oil Recovery & Fracking
And then there’s the biggie, getting more oil and gas out of tapped-out deposits. The CO2 is pumped out of the ground in one place where geological conditions have put a bunch of it, cleansed of sufficient water and impurities to be useful, then turned into supercritical CO2 and piped to the oil wells.
The USA is doing this far more than any other country, part of the reason it went from being an oil-starved country under the thumb of OPEC in the 1970s to the world’s biggest exporter of oil today. Yes, in case you weren’t aware, the USA produces more oil than Saudi Arabia, about 7 million barrels a day more, or about 50% above Saudi production.
Unsurprisingly, the USA has by far the largest network of CO2 pipelines, about 2,600 km of the global 3,000 km. The longest one is just over 800 km, or 500 miles.
Of the 230 million tons of CO2 that are used globally, about 90 million tons is used for enhanced oil extraction. Not exactly the kind of circular economy you normally think about, although ExxonMobil and others try to pretend it is. It’s a bit of a shell game to take CO2 out from underground in one place, pump it back underground in another, get a bunch of oil out which when used as intended turns into a lot more CO2, and then claim to be virtuous.
Carbon Sequestration
Sharp minds will have been thinking ahead and seeing what else the fossil fuel industry wants to use this for. That’s right, capturing CO2 from burning fossil fuels or the air, making it supercritical, piping it to some geological store under the sea or underground, and the pumping it down there to stay buried forever.
Will this work? Kind of. The engineering all works. The industry is already making about 50 million tons of supercritical CO2 in the USA, piping it hundreds of kilometers and putting it underground. That all works and is proven and demonstrated, which is part of the reason that the industry feels bad when people say it doesn’t work.
But let’s get back to some quantification. CO2 is a cheap commodity when it comes up from underground in large volumes, US$30 to $50 per ton. The fossil fuel industry doesn’t use electricity to power its compressors for the most part, but natural gas or oil. It still needs 90 kWh of energy, but it gets it from much less efficient fossil fuels. Heat energy to mechanical energy is in the 30% range, but nobody in the industry cared because they were using their own product in small amounts and it was cheap.
This is one of many places in the fossil fuel extraction, processing, refining, and distribution value stream where fossil fuels are burned to create even more greenhouse gases. Clearly that’s not suitable for any remotely virtuous carbon capture and sequestration, so they’ll either have to bolt on more carbon capture technologies or use electricity that could be employed for something with actual economic value instead.
But let’s do some more quantification. Let’s just take the 50 million annual tons over 2,500 kilometers of pipelines in the USA. That sounds like a lot, but it’s under 6,000 tons per hour in 2,500 km of dozens of pipelines.
Let’s pretend those 50 million tons are being turned into supercritical CO2 with electricity. What would that cost? At 90 kWh per ton and the US average industrial price of electricity in 2023 at just under 10 cents, that would turn into about $9 per ton or just under $450 million for the initial creation of the substance.
But wait a minute. One of the interesting properties of supercritical CO2 is that it can vary radically in density with fairly small changes in temperature or pressure. To keep pipelines from exploding, the contents have to be maintained above 31° Celsius and 74 atmospheres worth of pressure. This is worse than natural gas or oil pipelines where extra pumps are used to keep the stuff moving along, and if one fails all that happens is that movement is slower.
With a supercritical CO2 pipeline, compressor pumps are necessary to pour more energy into the fluid in order to keep its temperature and its pressure in the right zone. And because of that temperature sensitivity, the pipelines have to be insulated. More costs result.
Construction cost of a supercritical CO2 pipeline in the USA a decade ago was about $30 thousand per 2.5 centimeters of diameter per kilometer of distance traveled as a result. The average US pipeline is about 0.8 meters in diameter. The math suggests the US CO2 pipelines capital cost approached $24 billion. Amortized across a 30-year lifespan, that turns into about $16 extra per ton of CO2 on top of the $9 per ton for the basic manufacturing of supercritical CO2.
So we’re up to $25 dollars per ton cost without actually getting the CO2 from anywhere or putting it anywhere. Are there more costs? Yeah, those compressors are burning energy to maintain the CO2 in the supercritical temperature and pressure range. Given the requirement, it appears that booster stations might not exist on pipelines less than 100 km, but they certainly will on longer pipelines. Those compressors don’t have to put all of the energy in, but at least 10%.
At the US median length of 400 kilometers, that’s three booster stations or so, and another 30% energy requirements. Call it another $3 per ton of CO2. That’s $28 per ton of supercritical CO2 just for compressing it and transporting it. Never mind getting it or using it.
And those are short distances from large geological stores of CO2 to tapped-out oil wells. Those are also the costs of the lowest level of temperature and compression, and hence density. Want more density? Put in three times the energy and three times the energy cost as a result, bringing the total cost per ton potentially over $50.
Real Carbon Capture Distances & Scales
Of course, CO2 isn’t magically generated close to oil and gas wells except by the industry that extracts oil and gas. And it’s in nowhere near the concentrations as existing geological stores. And it’s 2-3 times the mass of the fossil fuels burned in its creation.
Let’s compare and contrast a bit. The USA has over 5 million kilometers of fossil fuel pipelines carrying crude oil, natural gas, gasoline, and diesel. Crude oil tends to be the biggest, often over a meter in diameter. Gasoline and diesel tend to be the smallest, perhaps 0.2 meters. The median is about half a meter.
Given the varying energy densities, with natural gas being lower density than normal CO2, never mind supercritical CO2, I won’t do more than napkin math on this. But getting supercritical CO2 up to the energy densities of oil, gasoline, and diesel requires either the $50 energy levels per ton, or pipelines that are some combination of a lot bigger in diameter or a lot more of them. Basic supercritical CO2 at 164 kg per cubic meter is about five times less dense than oil or coal.
Let’s give the fossil fuel network a break and assume that we take away half for redundancy of considering oil vs diesel and gasoline. And another half of the remainder for the lower density of natural gas. Then assume a reduction in fossil fuel consumption only to stationary point sources like oil and gas extraction facilities, oil and gas pipelines and coal, oil, and gas power stations. Call it 20% of demand. Then multiply it by 2.5 because of the 2-3 times the mass of CO2 as the fossil fuels burned in its creation. Oh, and then double it because the source of CO2 isn’t conveniently near the sequestration sites the way it is with enhanced oil recovery.
So we’re at under 10% of fossil fuels being used in this mythical carbon capture and sequestration end state levering the miracles of supercritical CO2. How much would just the pipelines and supercritical CO2 cost?
We would need about 25% of the 5.1 million kilometers of pipelines that have been built in the last 150 years in the country, or about 1.3 million kilometers. That’s about 500 times as much supercritical CO2 pipeline as exists today. Assuming the same ratio of costs as the current 2,600 km of pipeline, that would cost about $12 trillion to build. The operational costs would be in the same range. And no, current pipelines for other substances are not reusable for supercritical CO2.
All for something we can just avoid doing entirely by building renewables, transmission, and storage, and electrifying energy use.
By the way, this is all assuming dry CO2 which is non-corrosive, not wet CO2 which is very corrosive. As a result, add a lot of energy costs to remove water vapor from the CO2 before compression.
Dangers Of Supercritical CO2 Pipelines
Is there anything besides the absurd cost of building and operating pipelines for this nifty fluid/gas that would give us pause? Well, yes. If something goes wrong, like one of the booster compressors is unplugged or breaks, or someone accidentally runs a pickup truck into a pipeline, or accidentally punctures it with a backhoe, or a frost heave cracks it, or a rather large number of failure conditions, we’d better hope it’s where no people live.
When supercritical CO2 leaks, it turns rapidly into CO2, expanding a lot and turning very cold. The cold isn’t particularly a concern, but the gas itself is. You see, CO2 in its gaseous state is heavier than the air we breath. Until it mixes with normal air, it’s at a much higher concentration and much closer to pure.
The combination means that when there is a CO2 leak, it pools in lower areas. You know, like the dells and valleys a lot of people live in. Eventually it diffuses, but at the site of leak, there’s a problem.
Why? Well, humans can’t breathe CO2. And internal combustion cars which still dominate our roads need oxygen too. People pass out and potentially die. No one’s car will start to get them out of them danger zone unless they have a Tesla, and it won’t help unless they have Full Self Driving enabled and manage to set it before passing out. And emergency vehicles can’t get in.
Concentrations above 10% can cause convulsions, comas, and death. It can cause long-lasting organ and brain damage in survivors.
That sounds awfully alarmist, I’m sure. You’re saying to yourself, this guy is writing fantasy, not non-fiction.
Well, let me introduce you to Satartia, Mississipi. It’s a little village on the east side of the state. It was once thriving, and now just has a few hundred people in the area around it. And it has a supercritical CO2 pipeline running through it.
On Feb. 22, 2020, a sunny Saturday after a few weeks of rain, a perfectly typical landslide ruptured the pipeline. Yes, another failure condition. The supercritical CO2 inside of it basically exploded, because it turned from a dense liquid into a very non-dense gas pretty much immediately. That’s the same thing that happens in guns by the way, but we make it happen by igniting gunpowder. Ditto explosions. Big bang, plume of white smoke, startled people all around it.
It leaked CO2 for four hours before the operators shut it down.
What happened? 45 people unconscious and twitching on the ground, suffering from a lack of oxygen to the brain. Cars wouldn’t start. Over 200 evacuated. Emergency vehicles couldn’t get in because they shut down. $4 million costs.
No one knew what to do. Emergency responders had no idea what was going on.
And a very minimal approach to carbon capture and sequestration would put these pipelines everywhere in the USA, including all those places where a lot more people live. Right now, pipelines go from deeply rural areas to deeply rural areas, because existing geologically sequestered carbon is only extracted where people don’t live, and it’s used for enhanced oil recovery where people don’t live, and the land between is mostly empty too.
None of that is true if supercritical CO2 pipelines are used for carbon capture at places where lots of fossil fuels are burned. 500 times as much pipeline and CO2, a very great deal of it in highly populated areas.
There are a lot of reasons to think that carbon capture and sequestration is a very dumb idea. But the basics of transporting even a fraction of it with their associated costs and dangers should make it clear that it’s even dumber than most people think.
Nonetheless, the US government is spending a lot of money on this, as are governments with big fossil fuel royalties around the world.
Supercritical CO2 Power Generation
And now, the last category. There are hypothetical energy solutions the US DOE and others keep beating their heads against. One of them is perovskites for solar. Then there’s fusion. Another is concentrating solar power. And finally is using supercritical CO2 instead of water and steam in electricity generating turbines.
They share something in common. They’ve been promised as the next big thing in energy for decades. The DOE and other organizations keep making amazing sounding announcements about their potential. Millions and billions keep getting thrown at them. Credulous journalists who are STEM and economics illiterate write clickbait headlines and stories about them, including in CleanTechnica. None have ever panned out economically compared to much simpler alternatives.
They are all surplus to requirements. They are unnecessary. They are expensive, complicated, and have innumerable failure pathways. In a world with wind turbines and solar panels built by the millions and billions with massive global supply chains, we don’t need absurdly complicated and difficult things for energy. They are fun to research, I’m sure. They are fun to build prototypes of, I’m sure.
And so, supercritical CO2 generation. All this does is replace the working fluid and gas, water and steam, with CO2 and supercritical CO2. Like heat pumps and decaffeination of coffee, it’s a closed system. Not much CO2 is used and it stays inside the system. All excitement about this being a place where lots of CO2 will be used, in other words, is misplaced. It’s a tiny rounding error of CO2 use, even if it was massively commercialized.
Is it easy to do? No, no it’s not. One of the things I do when I assess technologies is see how long they’ve been around without being commercialized. The first prototype of a supercritical CO2 Brayton cycle generator was built in 1948. That’s 75 years ago. This technology is going to be 80 soon. It’s geriatric.
A rule of thumb is that if a technology is decades old and hasn’t been successfully commercialized despite a lot of attempts, it’s safe to bet that it probably never will be, at least not in any reasonable time for solving something like global warming.
Why? Well, corrosion, micropitting, extreme materials challenges, lots of failure conditions, and a host of other technical challenges have blocked useful movement. There are a couple of operational prototypes still, but really, they are laboratory kit, not something worth talking about after 80 years of failure. A simple Google search should keep credulous journalists from writing frothing prose about them, but no, that’s not the world we live in.
The same journalists are typically incredibly fascinated with hydrogen for energy, and perovskites, of course.
Thermal Generation Is Going Away
And thermal cycle electrical generation is diminishing rapidly, something the supercritical CO2 proponents and the STEM and economics illiterate journalists who gush about it seem to be missing. Coal plants are thermal generation. The USA has halved the number of them, as have other countries. They are going to disappear in the coming decades, so retrofitting them with expensive new CO2 boilers and turbines isn’t going to happen. Ditto nuclear plants, which are aging out and will be diminishing in number in the developed world in the coming years.
You can’t just drop a supercritical CO2 turbine into an existing coal or nuclear plant, you have to rebuild the entire thermal and generation cycle. No one is going to build a natural gas thermal plant with them because combined cycle gas plants are already as efficient as proposed supercritical CO2 plants and a lot simpler with fewer failure conditions.
Combined heat and power won’t be using thermal generation either. That’s going to be using heat pumps. Airports and campuses are ditching their natural gas co-generation units for geothermal heat pumps all over the place. No market for supercritical CO2 turbines there.
No retrofit market. The vast majority of new electrical generation capacity being wind and solar so virtually no potential new market. No new co-gen market.
What’s left? How about small modular nuclear reactors! They are a first of a kind, nonexistent and commercially non-viable product. They were tried and failed decades ago. Most of the technologies in them were developed decades ago and had no commercial successes for a variety of well documented reasons. Surely they could use an efficiency boost with another first of a kind technology. And, of course, the SMR proponents are all over that. But that’s like SMRs being used to make hydrogen for energy, bolting two technologies together to make something worse.
Be Super Critical Of Supercritical CO2 Hype
Supercritical CO2 is fascinating stuff. It’s a great solvent, it’s great for extracting useful compounds from plants, its much better for dry cleaning than what went before and it’s a great working fluid for heat pumps. There are a lot of small scale use cases for it that make perfect economic sense and are safe.
But by far the greatest user is the fossil fuel industry, and they want to multiply its use by orders of magnitude to perpetuate their business. They want to add a few percentage points of efficiency to burning fossil fuels for electricity instead of building wind turbines and solar panels. They want to pipe it through highly populated areas where pipelines will fail and kill people.
If you are a journalist who covers clean technology and have been writing clickbaity things about supercritical CO2 recently, give your head a shake. If you are a reader who likes reading about new tech, be much more skeptical about the substance and resist the clickbait.
Have a tip for CleanTechnica? Want to advertise? Want to suggest a guest for our CleanTech Talk podcast? Contact us here.
EV Obsession Daily!
I don’t like paywalls. You don’t like paywalls. Who likes paywalls? Here at CleanTechnica, we implemented a limited paywall for a while, but it always felt wrong — and it was always tough to decide what we should put behind there. In theory, your most exclusive and best content goes behind a paywall. But then fewer people read it!! So, we’ve decided to completely nix paywalls here at CleanTechnica. But…
Thank you!
Community Solar Benefits & Growth
CleanTechnica uses affiliate links. See our policy here.