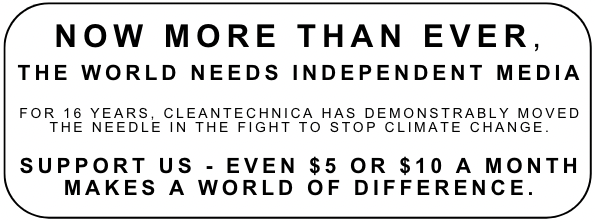
Sign up for daily news updates from CleanTechnica on email. Or follow us on Google News!
Key takeaways
- Early adopters of charging microgrids can gain a competitive edge with flexible pricing
- Solar-powered microgrids cut costs with zero marginal cost energy
- Collaboration on green corridors boosts savings and revenues
First movers with electric truck charging microgrids have a superpower, which is much more flexible energy pricing options to maximize profit. The big truck logistics firms that operate numerous depots, truck stop chains, and turn-key services vendors need to put on this cape.
As the authors — Rish Ghatikar and Michael Barnard, experts in sustainability, transportation, and strategy — explored in their diagnosis of the challenges of truck charging, there are overlapping concerns which can heavily slow down deployment of megawatt-scale charging solutions to accelerate truck electrification, and getting derailed into non-charging value propositions is one of them. The first action article dealt with creating standardized, incrementally increasing capacity microgrids to roll out consecutively to each site over several years. A related key lever is the electricity pricing flexibility that a charging microgrid with solar power provides.
Truck logistics firms and truck stop and depot operators have few levers to deal with the margin that they can make on the energy services that they rely on today. Wholesalers of diesel are trucking a fully commoditized product.
Upstream cost and price fluctuations can be exploited for short term increases in margin by truck stops, but just represent additional expenses for logistic firms. Every truck stop is price-aligned in local markets because competition is fierce. Margins on fuel sales today are in the 1% to 5% range, hence the captive market goods and services sales that have exploded at truck stops.
Locations with on-site refueling facilities typically meet specific operational and logistical criteria. These facilities are most common in depots supporting large fleets with high fuel consumption, where centralized refueling reduces costs and improves efficiency. Remote locations or urban areas with heavy traffic often necessitate on-site options to save time and ensure fuel availability. Additionally, bulk fuel purchasing and centralized monitoring of usage make on-site refueling appealing for cost control and operational oversight. Truck depots with tight schedules or integrated maintenance services often benefit from this setup, enabling seamless truck turnaround and compliance with regulatory safety standards.
The United States has over 577,000 registered motor carriers, with the majority of them operating a form of depot or facility for freight operations. Of the roughly estimated 15,000 major logistics depots with larger terminals in the country, perhaps a quarter have onsite refueling facilities today, per a rough estimate of the likelihood of factors converging to make it worthwhile by the authors. This comes with downsides, as onsite refueling typically creates the same environmental remediation challenge for the real estate as is found with public refueling stations, something that can prevent later resale and some rental use case for the property in the future.
For both truck stops and depots, the cost-benefit balance changes substantially when electrified trucking and charging infrastructure is considered.
Consider a hypothetical scenario for a large heavy-duty truck stop that can also charge light-duty vehicles (see figure 1 below). It has battery storage beside a restaurant, repair, and rest facilities providing 8 MWh of buffering storage energy. It has a refueling/charging canopy, rooftop and parking lot canopy solar charging covering eight acres in total, capable of generating 5.2 MW of electricity when the sun is shining, and will still generate some on cloudy days. It has 6 megawatt-scale truck chargers and perhaps 20 Level-3 light vehicle chargers under the solar canopies. It has a grid connection capable of providing 2 MW of power to the battery 24/7/365. (The state of charge of the battery in MWh isn’t shown, as the chart is already somewhat busy.)
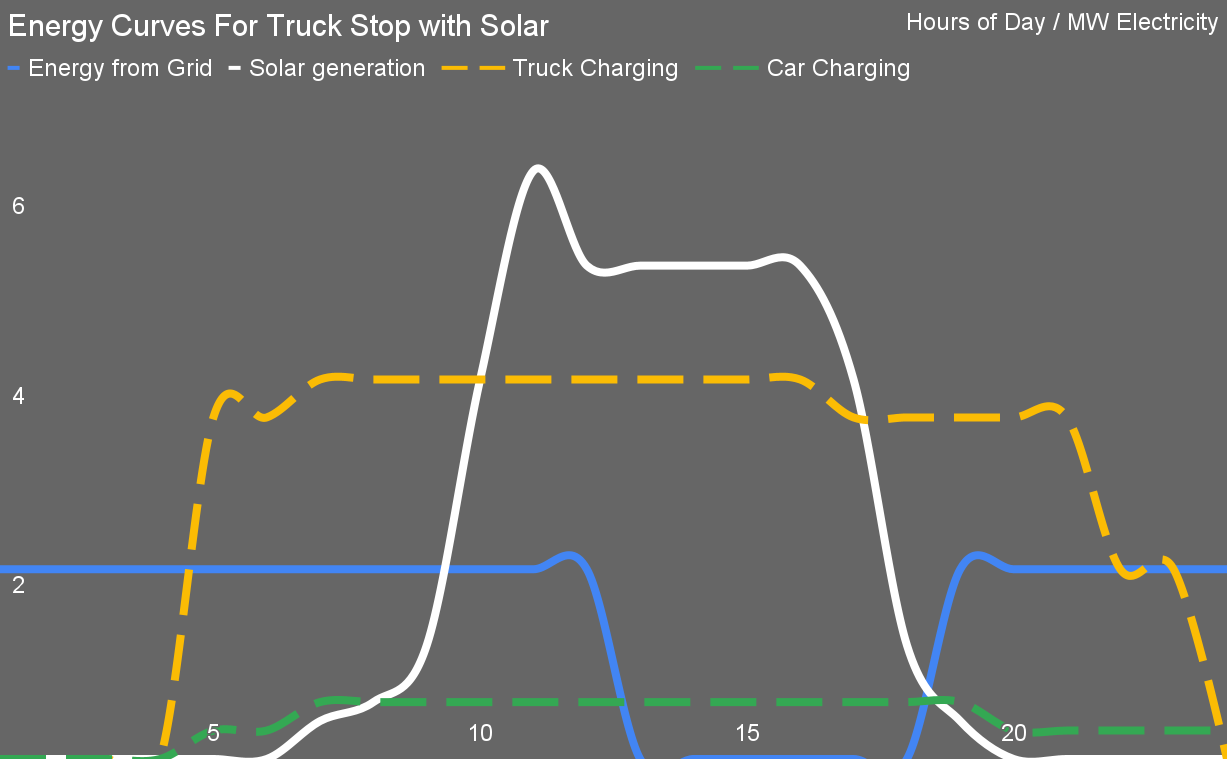
This configuration can charge around two hundred light duty vehicles and heavy duty trucks each per day, about 80 MWh of electricity. The solar panels, which have zero marginal cost of electricity and are inexpensive, commoditized 30-year assets with virtually no maintenance requirements, would provide a greater percentage of the charging electricity than would be drawn from the grid, roughly 60%. The grid connection would be much smaller and faster to deliver — while still taking a year or more — than attempting to charge this volume of vehicles from the grid alone. This increases the argument of availability and resiliency of electricity for heavy duty and medium duty vehicles.
The battery can be charged from the grid more in the lowest electricity rate hours or when the grid demand is low during the day. At present, this simplified energy scenario shows no grid draw when solar generation is at its peak, but it could equally be optimized for higher draw at low electricity costs during solar peaks, something that modeling will refine. At night it can charge up to optimum levels for expected daytime demand and supply. During the mid-day solar depressed electricity pricing regime which is already being experienced in multiple jurisdictions, the batteries can be filled for the evening charging period when grid electricity rates and/or the axiomatic grid peak demands are high.
Computerized electricity management systems can juggle the equation of grid electricity price, projected charging demand, projected solar supply, and battery performance to get the lowest possible cost of electricity provided to trucks every day. It will still service approximately 200 heavy and light vehicles each daily. This is another operational revenue that the truck stop operators and heavy and light duty vehicle owners can uniquely benefit from.
Because the solar electricity supply has zero marginal cost to the truck stop, with only the capital costs to service, the truck stop operator has significant flexibility in retail pricing of electricity and its operational use.
One key factor in pricing consideration models for electrification is still the average cost of diesel. All potential price points should be below the energy cost per mile of diesel trucks to strategically create more value to electrified customers. The margin on sales of electricity will still be high, but price gouging is not a strategically wise move, as pushing other competitive truck stops that don’t electrify as quickly out of business is a design point of the strategy.
In the scenario the authors envisage, the truck stop chain would centralize this power management and provide it as a service to local truck stop managers. Shrewd turnkey services contractors building truck stops would provide the same service.
When 60% of the fuel a truck stop provides every day is essentially free, the flexibility for profit maximization is high, especially in early days when they are the only game in town. Over time as more electrified truck stops emerge, the competitive landscape will change, but in the initial rush, early movers that can build incrementally scaled microgrids will be able to expand their market share.
The scenario is different for major logistics depots (illustrated below in figure 2). The same grid connection and the same solar generation again will service roughly 200 heavy duty and light duty vehicles each day. In this model, due to lower travel distances per vehicle due to a more local and regional duty cycle, two-thirds of all electricity in this scenario has zero marginal cost to the logistics firm. (Similar to figure 1, battery state of charge is excluded to reduce the busy-ness of the visualization.)
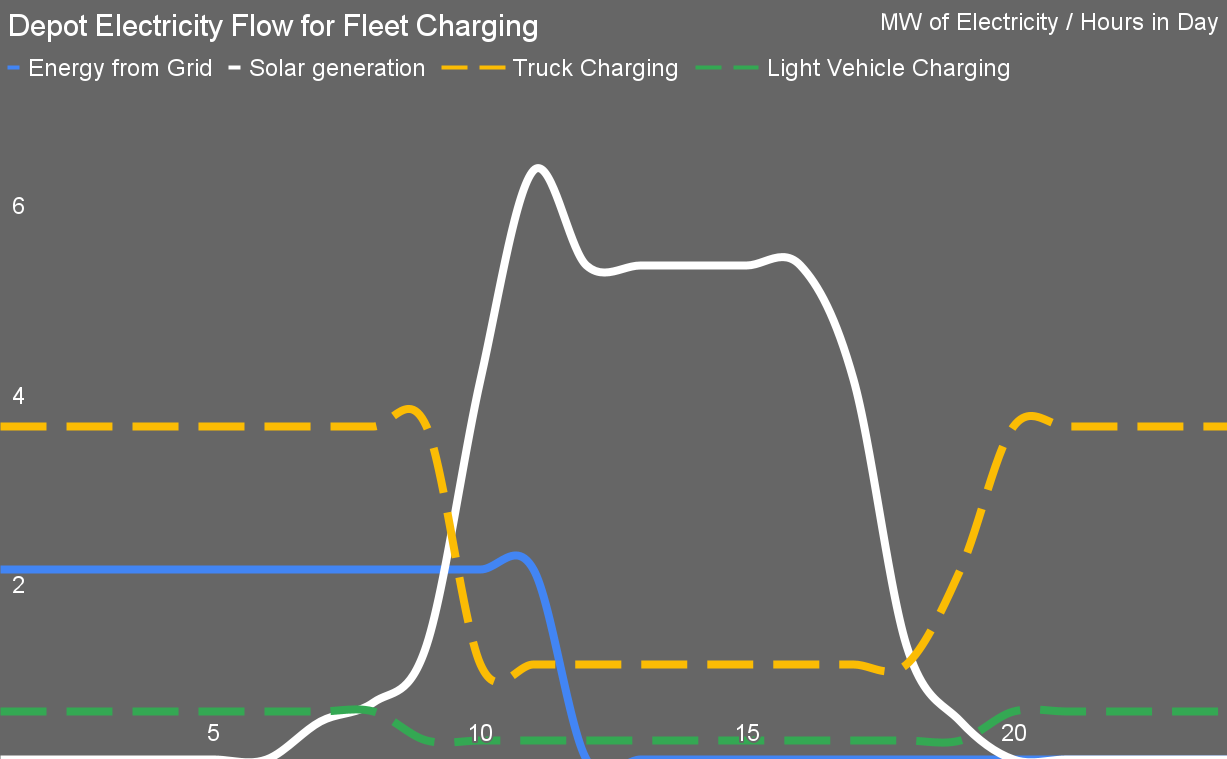
However, the requirement for megawatt-scale chargers and power equipment is optimally sized and replaced with many more Level 3 and Level 2 chargers. Each vehicle’s dominant pattern is overnight charging in this scenario, with much lower levels of electricity flowing into each one at any given time over a longer duration. Further, depot fleet vehicles, on average, travel fewer kilometers per day and can increasingly depend on en route megawatt-scale charging for longer journeys. The buffering battery must be scaled up for this and the megawatt-scale chargers on site are replaced with many more Level 3 and Level 2 chargers.
One key challenge that has to be designed into the truck fleets and charging solution is that standardized megawatt-scale charging adaptors are different from present Level 2 and 3 charging adaptors, so fleet charging and flexibility may be inhibited depending on the vehicle manufacturer if they don’t support both. This challenge will not be relevant when projects deploy the North American Charging Standard chargers and trucks support them.
At this point, the profit maximization model looks at each vehicle’s expected route for the following day with contingency, and optimizes the amount of electricity it receives for that journey. It’s unlikely that it will ever be more profitable to charge at retail locations barring exceptionally long routes. Over the night hours, a Pareto optimum can be identified for the size of the grid connection vs the size of the buffering battery for the use cases for the depot. More optimization can occur with operational data analyses between depot and logistics operators.
Once again, building to this point is an incremental process with this degree of electrification being the third or fourth increment. Early increments will optimize lower revenue against lower capital costs and be constrained by grid connection timeframes.
Organizations considering this will quickly realize that the solar generation curves in the examples above are for a notional average day in spring or fall. On longer summer days, more solar power will be generated, more than the buffering batteries and vehicles can absorb. Almost every utility in the US has net metering in place, where local power generators can put electricity they don’t require into the grid and receive compensation for it. The scenarios above have electricity from the grid going to zero for much of the day, allowing for zero marginal cost fuel. However, electricity flowing back into the grid provides another revenue lever.
But the larger concern with this average is not long, sunny days in July, but short, dark days in December. The solar curves will be much lower, but trucks will still have to roll. Thankfully, there are three easy answers. Upscaling the battery capacity and solar by 10% each covers 40% more of the declining curve of days outside of the sweet spot. During the microgrid increments design process, modeling a dozen sites around the US against historical weather data per day — available on an hourly basis from the National Oceanic and Atmospheric Administration with historical records stretching back years — will enable optimization of each increment for scale and charging cost and benefits.
After those two levers are pulled, however, there will still be days without sufficient electricity for the needs of trucks. What’s the answer there for mission-critical sites beyond a bigger grid connection that delays all charging? Diesel, in two ways. For such selective depots and truck stops, diesel will remain a part of the truck energy equation for a couple of decades. Depots and truck stops already have backup generators intended for emergency use. During the modeling exercise, the days with insufficient solar generation and grid supply for the expected charging demand and what the electricity shortfall is will be clear. Running the backup generator to charge the buffering batteries on those days can be modeled. In many cases, the existing backup generator will be completely adequate. In others, part of microgrid deployment will be increasing the size of the generator at some sites.
Doesn’t this defeat the purpose?, many will ask. No. First, if trucks are running on solar-generated electricity and increasingly low carbon grid electricity 95% of the year, running them on diesel-generated electricity for 5% of the year is still a major reduction in emissions, which is a significant part of the point. Second, an electric truck running on diesel-generated electricity is actually lower emissions than a diesel truck due to both the generator and the truck operating at optimal efficiency. Depot operators also have the opportunity to keep a few of their diesel trucks around on much lower duty cycles, using them on those dark December days, if that’s the optimal solution.
Depots that electrify charging with solar and battery buffering isolate themselves from fluctuations in both diesel prices and grid electricity prices, and have 66% zero marginal cost energy for moving goods. This gives them a strategic pricing advantage over legacy depots that don’t have the ability to invest, enabling early movers to take business away. One of the authors, Barnard, was discussing this strategic disruption coming to the road freight industry with a freight forwarder in Brussels recently, who projects 10% to 20% expense reductions for battery-electric trucking operators, and hence a very different competitive landscape.
The major logistics firms that take advantage of this integrated microgrid charging strategy early will be about to outcompete the ones that don’t, taking market share during the transformation.
Vehicle grid integration services facilitate interaction between electric trucks, microgrid infrastructure, and the electric grid to enhance grid reliability and integrate renewable energy. These services include managing charging based on grid prices or demand, using parked trucks (e.g., depots) to power local infrastructure, and powering the grid, when the freight operations allow it. Ghatikar has focused on improving vehicle grid integration operational economics and enabling interoperability of transportation systems across the grid stakeholders from standards. The standards cover data and communications, charging controls, and bidirectional energy flow, enabling trucks to be ready to offer vehicle grid integration services. Key standards include those developed by organizations such as SAE International, Open Charge Alliance, OpenADR Alliance, IEC, IEEE, etc., which address the technical specifications for power systems’ connectivity. Standards for these services help cost-effective management of peak loads, grid reliability, and smart charging that aligns with integrating renewable energy sources into microgrids and managing grid reliability, and thus increasing the certainty of revenue streams while providing environmental and social values.
For both logistics firms and truck stop or depot operators, solar- and battery-enabled microgrids with dynamic energy management open up new pricing and competitive levers to provide high-grounds. This gives them the opportunity to overcome part of the challenges of capital expenses preceding revenue and uncertain revenue streams identified in the diagnosis section of this self-reinforcing strategy.
For green corridors, the differences in charging vs sunshine suggest that it would be in the best interests of both truck stop operators and logistics firms to compare notes to optimize costs vs revenue. There’s the possibility to reduce the battery and solar arrays at depots, increasing dependence on en route charging at microgrid-enabled truck stops, at a lower total cost of ownership for the logistics firm and greater revenue for the trucking firms.
For all three audiences, as initiators, starting to explore their strategies around microgrid truck charging and developing revenue models that accommodate the changes becomes important.
Previous articles in this series:
About the authors:
Rish Ghatikar has an extensive background in decarbonization, specializing in electric vehicles (EVs), grid integration, and demand response (DR) technologies. At General Motors (GM), he advanced transportation electrification energy services, as part of a broader climate strategy. Previously, at Electric Power Research Institute (EPRI), he focused on digitalizing the electric sector, while at Greenlots, he commercialized EV-grid and energy storage solutions. His work at the DOE’s Lawrence Berkeley National Laboratory spearheaded DR automation to support dynamic utility pricing policies. An active climate advocate, Ghatikar advises on policies and technologies that align the grid with transportation and energy use for sustainable growth.
Michael Barnard, a climate futurist and chief strategist at The Future Is Electric (TFIE), advises executives, boards, and investors on long-term decarbonization strategies, projecting scenarios 40 to 80 years into the future. His work spans industries from transportation and agriculture to heavy industry, advocating for total electrification and renewable energy expansion. Barnard, also a co-founder of Trace Intercept and an Advisory Board member for electric aviation startup FLIMAX, contributes regularly to climate discourse as a writer and host of the Redefining Energy – Tech podcast. His perspectives emphasize practical solutions rooted in physics, economics, and human behavior, aiming to accelerate the transition to a sustainable future.

Chip in a few dollars a month to help support independent cleantech coverage that helps to accelerate the cleantech revolution!
Have a tip for CleanTechnica? Want to advertise? Want to suggest a guest for our CleanTech Talk podcast? Contact us here.
Sign up for our daily newsletter for 15 new cleantech stories a day. Or sign up for our weekly one if daily is too frequent.
CleanTechnica uses affiliate links. See our policy here.
CleanTechnica’s Comment Policy